I want to describe a certain beautiful experiment, perhaps the most beautiful experiment in science. This is an experiment that has captivated me from the time that I first heard about it in high school. That’s because it’s simple to understand, and yet it captures the essence of what is truly messed up about quantum mechanics. This is a tale of two slits. And it would be no exaggeration to say that through these slits, we encounter a word that is so strange, it is beyond our human capacity to imagine.
The story is about the nature of light and matter. And it is driven by a fervent battle of ideas between some of the greatest minds in science. It begins at the turn of the eighteenth century.
By then, Isaac Newton had already made a name for himself as the biggest badass in science. He invented calculus (edit: although the origins of calculus are somewhat mired in controversy), devised the law of gravity and formulated the laws that govern how things move. That’s pretty eventful for a few decades (in fact, he did much of this work in a single year), and it’s almost inhuman that all this came from a single person.
And things were just getting started. By the turn of the century, Newton had turned his considerable attention towards the problem of light. How does it work? What is it made of? Using a series of simple, methodical experiments, he argued that if you stripped light down to its tiniest constituents, you would end up with particles that he called corpuscles. This idea was widely adopted, and became the mainstream scientific opinion for over a hundred years.
There were always doubters to this idea, but they weren’t many of them, and they weren’t popular. It was another brilliant English scientist, Thomas Young, who would take the next step in understanding light.
Young was quite the Renaissance man. In addition to being a physicist, he made significant contributions to fields as diverse as music, language (he compared the vocabulary and grammar of 400 different languages), Egyptology (he partly deciphered Egyptian hieroglyphics from the Rosetta stone) and the physiology of vision.
But what Young considered his greatest achievement (and he had a few) was overthrowing Newton’s century-old notions of light. In its place, he argued that light was not made up of particles, but was instead a wave, quite like the ripples on the surface of water.
At first, he met with huge resistance to his ideas. But in 1803, Young convinced his skeptics with a simple, game-changing experiment.
Here is how it works. Imagine light is composed of tiny particles, like droplets of water, that are being spit out from a lamp. If this light falls on a barrier that has two thin slits sliced into it, it will shine through these slits. If this ‘garden hose’ theory of light is correct, you would expect to see something like the following picture.
What you see here is the drops passing through the slits and striking the wall in basically two places. At the right is a plot of how many drops hits the wall, and there are two piles of water drops that are directly behind the two slits.
Now imagine the same experimental setup, but instead of spraying droplets, we fill the room ankle-deep in water. Things now look something like this:
At one end, you start sending out ripples in the water. Just like when you drop a pebble into a pond, these ripples move rhythmically outwards in circles, peak followed by trough followed by peak.. and so on. When the ripple hits the barrier, you now have two ripples coming out, one from each slit.

And as these ripples start moving towards the screen on the right, something entirely new happens – they interfere. That is, there are places on the screen where the crest of one wave hits the trough of another, and the two waves cancel each other out. And there are other places where two peaks or the two troughs line up – the waves reinforce. If you were to look along the screen, you would find places where the water stays completely still, next to places where it splashes wildly.
If you wanted, you could do the same experiment with sound. Instead of slits, you could have two speakers. Sound is a wave, it’s a vibration in the air that wiggles our eardrums. As you move your ear along the screen, you would find places where the two sound waves reinforce, and you would hear a louder sound. And there would be also places where you would hear nothing as the sound waves cancel each other out (no vibration). The overall picture you get is this striped interference pattern shown above, of alternating highs and lows.
So Young performed this experiment with light. To everyone’s surprise (but his), he found that light doesn’t act like the bullets of a machine gun. What he saw on the screen was an interference pattern – alternating bands of light and dark. The interpretation was unambiguous – light behaves like a wave, not like a bunch of particles.
And for the next century, the wave theory reigned supreme, until no less a figure than Albert Einstein came onto the scene. In his amazing year 1905, Einstein explained a famous experiment – the photoelectric effect – by invoking the idea that light is made of particles that carry energy. He would later win the Nobel Prize for this achievement. Somewhat embarrassed by Newton’s corpuscles, physicists rebranded these particles with a new name – photons.
And soon after, engineers were building devices that could make noises whenever they detected light. Rather than hearing some kind of continuous splish-splosh that you may expect from a wave, they would hear a sound like individual raindrops – tick, tick, tick. Each of those ticks was a photon striking the detector.
Now, if you’re with me so far, this is a point where you can stop and scratch your head. On the one hand, Young proved that light is a wave. But then you have Einstein and these detectors. They’re practically screaming in our ears that light is a particle. So what’s really going on here?
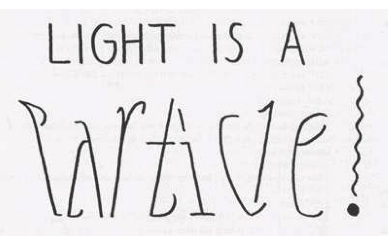
This is the dilemma that gave rise to quantum mechanics – depending on what experiment you do, light seems to behave like a wave, or like a particle. It turns out, as physicists later discovered, that this is true for any kind of stuff, not just light. If you take atoms or electrons and send them through the double slit, they’ll behave like the interfering ripples, not like water drops.
My teachers made a big deal about this in high school, and I used to always wonder what the fuss is all about. After all, water ripples are waves, but water is made of particles (H20 molecules). No one talks about the wave-particle duality of water. What’s different about these subatomic particles?
Well, I’m going to tell you now what my school teachers never taught me, but I learned from popular science books instead. Let’s say you’re a rogue physicist, and you think that everyone’s having you on with all this wave-particle mumbo jumbo. Here’s a simple experiment you can perform to prove them wrong. What if you repeat the double slit experiment, with one small change – this time, you send stuff in one particle at a time. Before you read on, take a minute to think about what you would expect to see on the screen.
If you haven’t come across this before, then your intuition is probably telling you that the particle couldn’t possible interfere, because there aren’t any other particles around to bump into.
Now, here’s what actually happens. Remember, at any given time there is only one particle in the picture. (the video you’re seeing was done for electrons, but the same thing happens for light)
How wild is that? Our rogue physicist’s idea fell flat. Even though you send in the electrons one at a time, they still manage to interfere. If they behaved like billiard balls, they would be going through one slit or the other, in which case you would just see two bright bands that are behind the slits. Instead they produce these alternating dark and light bands. How could this be?
“Aha!”, says the rogue physicist. “I’ve figured out what must be happening. The reason I was thwarted is because I must have been wrong about the electron in the first place.”
“Maybe what’s really happening is that the electron somehow splits into two pieces, goes through both slits, and then interferes with itself.” And so the physicist hatches a plan to vindicate their idea.. by looking to see which slit the electron really goes through.
And here comes the mind-bogglingly bizarre thing about quantum mechanics. Let’s say you shine light on the electron to see which slit it goes through. What you see is that it always goes through one slit or the other, nothing weird is going on. But, once you do this, the interference pattern disappears and instead you get just the two bands!
If you detect which slit the electron goes through, then it behaves like a plain-old billiard ball. But when you turn off the light, and can’t tell which door the electron goes through, it interferes and you get the pattern of light and dark fringes. So, just by looking at the particles, we are changing the outcome of the experiment.
There is no picture that you can hold in your head that will explain these results. It’s not like a billiard ball with some strange gears and clockwork inside it. It’s something fundamentally different.
But they have gotten used to the idea that measurements matter. In the quantum world, a measurement fundamentally alters the thing that you are studying.
Rather than finding this strangeness unsatisfying, I find it incredibly exciting. In a way, I feel that we’ve been pretty lucky so far. Our brains have evolved to understand the African jungles and savannah in which we grew up. Everything that makes sense to us is not too alien from this environment. But when we start to push our understanding to this sub-atomic level, there is no good reason for our macroscopic intuition to apply here. Like Alice’s looking glass, these two slits are our door into a strange new world.
And if you thought things couldn’t get any weirder, you’re wrong. Here’s the clincher. Let’s say you repeat the double slit experiment, but this time you put a detector only behind one slit. So I know if the electron goes through slit B, but not if it goes through slit A. What would you expect to happen now?
What happens is not that you get half an interference pattern. Instead, this is no different from the previous experiment with two detectors. The electrons pile up behind the slits.
Think about what this means. If you are still clinging in your head to a picture of an electron as a ball flying through one of the slits, then you are forced to conclude that the electron going through slit A somehow knew about the detector in slit B, and decided not to interfere. This is absurd (even by quantum standards) and many a crackpot have travelled down this road. Instead, what we should learn from this experiment is that our classical picture is fundamentally broken. The electron does not behave like a ball, or anything else we can readily imagine.
Well, then, what the hell is it? To find out, tune in to part 2 of a tale of two slits.
That’s it for now. I’ll let this madness settle in. In part 2 of this post, I will explain the rules of the new game in town: quantum mechanics. I’ll revisit the double slit experiment, but this time I’ll describe the elegant methods that physicists use to make predictions in the quantum world. And I’ll comment on an interesting new experiment that revisits the double slit.
References:
If you want to understand how quantum mechanics works without getting into the mathematical details, you could do no better than read Feynman on the subject. His book QED: The Strange Theory of Light and Matter has my vote for one of the finest popular science books of all time.
Richard P. Feynman (1988). QED: The Strange Theory of Light and Matter Princeton University Press ISBN-13: 978-0691024172
Young, T. (1804). The Bakerian Lecture: Experiments and Calculations Relative to Physical Optics Philosophical Transactions of the Royal Society of London, 94, 1-16 DOI: 10.1098/rstl.1804.0001
The experiment that I mentioned with a detector behind just one slit is a special case of the Quantum Eraser experimental setup.
Here’s the writeup by Hitachi that goes along with the video embedded above.
After being very pleased with myself for coming up with ‘a tale of two slits’, I googled it to find that it had already been coined. However, my mild annoyance quickly turned to delight as I started reading Ethan Siegel’s excellent and entertaining blog.
Edit: Richard Dawkins has a way with words. In this fascinating TED talk from 2005 (one of the first talks on TED.com), he speaks eloquently about the idea that our brains have evolved to find the universe intuitive at a given middle-sized scale. He calls this our Middle World, and we can step out of middle world by looking at things that are at a different scales in size and speed.
Footnotes:
I remember that the double slit experiment, one photon at a time, is the first experiment I ever did in college. It was my first semester, and at the time, I didn’t expect it to work. It just seemed too far out to be true. Sitting in that dark room watching the interference pattern slowly build up on the computer screen essentially shook the classical physics out of me.